High-quality total RNA extraction from early-stage lamprey embryos
Abstract
High-purity total RNA extraction from animal embryos is essential for transcriptome analyses. lampreys, together with hagfish, are the only extant jawless vertebrates or cyclostomes and are thus key organisms for EvoDevo studies. However, extracting uncontaminated RNA from early-stage embryos remains challenging. RNA does not bind to the silica membrane in filter-based extractions, significantly reducing yields; and ethanol/isopropanol precipitation methods lead to contaminants, bringing down the optical density (OD) 260/280 ratio. The RNA extraction protocol was modified using precentrifugation and adding salts before isopropanol precipitation. This modification significantly increased RNA yield, removed contaminants and improved RNA integrity. Egg membrane sources were suspected to cause RNA purification problems because low-quality extraction does not occur in posthatching embryos.
METHOD SUMMARY
Lamprey embryos were homogenized in a solution containing guanidine thiocyanate and phenol, then centrifuged to remove surface lipids and precipitates including egg membrane fragments. After chloroform extraction, salt was added to the remaining solution before isopropanol precipitation to prevent coprecipitation with contaminants, resulting in highly pure total RNA extraction. This technique is particularly effective for early-stage embryos from single-cell to prehatching stages.
Obtaining high-purity total RNA from animal tissues is important for next-generation sequencing (NGS)-based transcriptomics and high yields of RNA are also crucial for single-cell RNA-seq analyses. Lampreys are one of two extant jawless vertebrates (cyclostomes) that, along with hagfish, diverged from gnathostomes in the earliest period of vertebrate evolution. Therefore, the early evolutionary changes of the vertebrate body plan can be explored by comparing cyclostomes to gnathostomes [1]. Lampreys are not laboratory animals, such as mice or zebrafish, but obtaining their eggs is relatively easy. In the mating season (in the northern hemisphere, March–June), more than 10,000 eggs can be obtained from a single mature female via artificial spawning [2]. Embryos can be raised in the laboratory in plastic dishes with 10% Steinberg's solution until the ammocoetes larval stage (Figure 1A). Eggs initially have an adhesive gel mass on the vegetal side of the egg membrane surface, which anchors them to the sand at the river bottom and then the gel mass disappears during the early cleavage stage [3]. Additionally, lamprey eggs have a double chorion [4], allowing them to be collected in 2-ml tubes for RNA extraction and for water to be removed without destroying them (Figure 1B). Hatching enzymes then weaken the egg membrane before hatching, which occurs at Tahara's stage 24: the midpharyngeal stage (Figure 1G) [3]. Lamprey embryonic cells contain an abundance of yolk granules composed of the lipovitellin–phosvitin lipid–protein complex [5]. These yolk granules are consumed during embryonic development but can still be found in the endodermal cells of posthatched larvae.
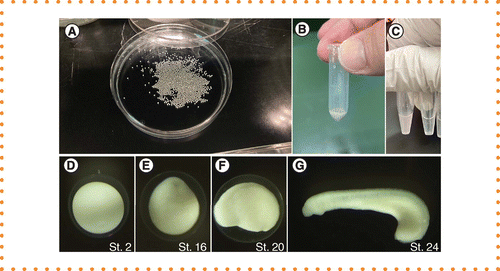
(A) Hundreds of eggs in a 9-cm dish. (B) 100 embryos collected in a 2-ml microtube. Embryos are surrounded by a double chorion; therefore, water can be almost completely removed without damage using a micropipette. (C) After isopropanol addition, solution becomes cloudy as per normal protocol (left tube), but transparent in this modified protocol (right tube). (D–G)Lethenteron camtschaticum embryos. Stage 2 (1 cell, D), stage 16 (gastrula, E), stage 20 (just after neural tube closure, F) and stage 24 (midpharyngula, G). Note that hatching occurs after stage 24.
Two major protocols derived from the acid guanidinium thiocyanate-phenol-chloroform (APGC) extraction method are generally used for total RNA extraction from cells or tissues [6]. The first involves centrifugation with isopropanol after separating protein/DNA and RNA using a solution containing guanidine thiocyanate and acid phenol (including the commercially available TRIzol reagent [Thermo Fisher, MA, USA], ISOGEN [Nippon Gene, Japan] and QIAzol [QIAGEN, MD, USA]). The second protocol uses a silica membrane to bind RNA in the presence of chaotropic ions, following the same removal protein and DNA as the first protocol (commercial kits include the RNeasy kit, QIAGEN).
However, neither of these protocols works well for extracting RNA from early-stage lamprey embryos. The isopropanol precipitation method has abundant contamination in the final RNA solution, and low optical density (OD) 260/280 and 260/230 ratios (<1.5 and <1.0, respectively). These contaminants cannot be removed by extra phenol/chloroform extraction or additional alcohol precipitation. In addition, the RNA integrity number (RIN) value of RNA extracted from early-stage embryos tends to be low with this method [7]. For transcriptome analysis of mRNA, it is generally necessary to use total RNA with a RIN score >8 to avoid bias toward the 3′ end region of the mRNA (see TruSeq Stranded mRNA reference guide, Illumina®). In contrast, the method using silica filters yields significantly less RNA (e.g., <10 ng/100 eggs). This may be due to contaminants preventing the binding of RNA molecules to silica. To date, there have been very few published studies on transcriptome analysis using early-stage lamprey embryos [8]. Additionally, while single-cell transcriptome analysis has been reported for the spinal cord and brain of ammocoete larvae [9,10], to our knowledge, there are none utilizing early-stage embryos. This may be attributed to the challenges associated with extracting RNA from early-stage embryos of lamprey with high quality and high efficiency. To overcome these problems, the QIAzol/isopropanol precipitation protocol was modified to improve the yield and purity of extracted total RNA as follows (step-by-step procedure available in Supplementary Appendix 1).
Embryos at different stages (stages 2, 16, 20 and 24; Figure 1D–G) of the Arctic lamprey, Lethenteron camtschaticum, were collected in 2-ml microtubes (Figure 1B, up to 500 μl) and homogenized with QIAzol reagent and stainless-steel beads. The homogenized solution was divided equally into three groups to evaluate the protocol. The first group was treated using the conventional APGC method (called ‘Normal’ in Figures and Tables) following the QIAzol Lysis Reagent instruction manual (QIAGEN), the second using a silica membrane filter according to RNeasy Lipid Tissue Mini Kit (QIAGEN), and the third group was treated using the modified precentrifugation and salt addition protocol (Supplementary Appendix 1).
Briefly, the modified protocol began with centrifuging the homogenized solution at 12,000 g for 15 min at 4°C and then transferring the solution while avoiding the lipids floating on the surface and the precipitates. Chloroform was then added to the solution, followed by 250 μl of a mixed salt solution composed of 0.8 M sodium citrate and 0.2 M sodium chloride for every 1 ml of QIAzol solution, and then half the usual volume of isopropanol (250 μl for every 1 ml of QIAzol). Although the solution becomes cloudy at this step in the normal protocol, it remains transparent in this protocol (Figure 1D), suggesting that precipitation is impeded by the high concentration of salts. The subsequent steps follow the normal protocol, with the final elution in RNase-free water. The results of the three protocols are summarized in Table 1. The normal precipitation method produced the highest amount of total RNA, but the absorbance peak shifted from 260 nm with a large peak observed at approximately 230 nm, resulting in extremely low 260/280 and 260/230 ratios, indicating contamination by proteins and other substances (Figure 2). Conversely, the RNeasy kit produced significantly lower yields at stages 2, 16 and 20 (Table 1). Bioanalyzer analysis showed that the RNeasy samples do not produce an rRNA peak until stage 20, resulting in an unmeasurable RIN (Figure 3). The modified protocol, with precentrifugation and the addition of salt, greatly improved the total RNA yield and quality (Table 1). Moreover, the highest RIN values, indicating RNA integrity, were obtained by using the modified method. As mentioned, high RIN values are crucial to obtain unbiased results in transcriptome analysis. This method yields significantly higher RNA recovery than the filter-based method, making it applicable for RNA extraction in single-cell analysis. Therefore, this method is expected to be useful for future single-cell transcriptome analysis in lamprey embryos.
Stage | NanoDrop | Bioanalyzer | ||||
---|---|---|---|---|---|---|
ng/ul | 260/280 | 260/230 | ng/ul | RIN | ||
2 | RNeasy | 5.92 | 0.83 | 0.14 | 7 | N/A |
Normal | 149.75 | 1.37 | 0.40 | 143 | 7.50 | |
Salt + precent | 62.54 | 1.87 | 1.68 | 106 | 8.80 | |
16 | RNeasy | 7.16 | 0.94 | 0.16 | 2 | N/A |
Normal | 122.23 | 1.39 | 0.30 | 146 | 8.90 | |
Salt + precent | 54.42 | 1.90 | 1.01 | 112 | 9.30 | |
20 | RNeasy | 6.63 | 0.89 | 0.15 | 6 | N/A |
Normal | 241.74 | 1.53 | 0.50 | 333 | 9.80 | |
Salt + precent | 109.75 | 1.98 | 1.61 | 243 | 9.80 | |
24 | RNeasy | 134.22 | 1.92 | 0.72 | 167 | 10.00 |
Normal | 832.99 | 1.90 | 0.78 | 1090 | 10.00 | |
Salt + precent | 661.92 | 2.09 | 2.14 | 1047 | 10.00 |

Vertical axes indicate 10-mm absorbance and horizontal axes indicate wavelengths (220–350 nm). Strong absorbances were observed around 230 nm and 260 nm peaks, and shifted right in RNeasy and normal protocols (except for stage 24), suggesting strong contamination, including by proteins. Note that input sample volumes for each stage were the same, but differed between stages.

Vertical axes indicate fluorescence units and horizontal axes indicate RNA lengths. RIN is a measure of integrity of purified RNA. RIN ranges from 1 to 10, with 10 being most intact. Total RNA with RIN ≥8 is generally considered to indicate acceptable integrity for RNA-seq. Highest two peaks indicate 18S and 28S ribosomal RNAs. RIN values for total RNA were improved when utilizing precentrifugation and salt addition, but RNA <200 bp was decreased. Note that input sample volumes for each stage were the same but differed between stages.
RIN: RNA integrity number.
The protocol for alcohol precipitation in the presence of high amounts of salts was used for total RNA extraction from plants containing proteoglycans and polysaccharides and RNA extraction from mouse liver, where these substances are abundant [11]. As shown here, this method can also improve the purity and yield of extractions from early-stage lamprey embryos. However, bioanalyzer results show that very little RNA of less than 200 bp was extracted using this method (Figure 3) and thus, the method should be avoided in small RNA research. In the presence of chaotropic salts, DNA or RNA can form hydrogen bonds with the -OH on the silica membrane. The reason RNA from lamprey eggs are not extracted by conventional methods (e.g., RNeasy kit) was likely due to the interference of contaminants that are not removed by guanidine-phenol extraction and subsequently inhibited the binding between RNA and silica in the following steps. These contaminants were present in the final product obtained by precipitation methods and inhibited RNA binding in filter-based methods. Although we did not investigate the identity or origin of these contaminants or the specific region of the egg from which they originated, it is certain that their influence decreases as development progresses, as shown in the Figure 2. Although we have not investigated the identity of the substance responsible for contaminants or its specific origin within the egg, it is evident from the results that the level of contamination decreases as development proceeds (Figure 2). Interestingly, at stage 24, RNA can be extracted successfully by any of these methods. Indeed, the reduction of yolk granules in the cells throughout development might also decrease the amount of lipids during stages 20–24, but the most significant developmental change during this period is hatching [3]. Thus, it is highly likely that the contaminants in the early stages are derived from the egg membrane. Additionally, the presence of highly adhesive substances that facilitate adherence of the egg to the river sand and double chorions may also impede RNA extraction. Although the manual removal of egg membranes from hundreds of eggs using forceps has the potential to reduce contamination, it is not a practical procedure during the rapid early stages of embryonic development in lampreys. Thus, in the case of early lamprey embryos, this new method is considered the most effective solution. This study was conducted only on the Arctic lamprey, L. camtschaticum, but is probably applicable to all other lamprey species since they also spawn at the river bottom in the same manner.
Supplementary data
To view the supplementary data that accompany this paper please visit the journal website at: www.future-science.com/doi/suppl/10.2144/btn-2023-0004
Author contributions
F Sugahara and J Pascual-Anaya conceived the project and performed the experiments. F Sugahara wrote the paper. Both authors discussed the results and contributed to the final version of the manuscript.
Acknowledgments
The authors would like to thank members of the RIKEN aquarium for housing and maintaining adult lampreys, and R Kusakabe, W Takagi, S Kuratani, H Nagashima and N Sato for lamprey sampling.
Financial & competing interests disclosure
This project was supported by RIKEN, Kobe, Japan, and the Japan Society for the Promotion of Science (grant numbers: 19K06794 and 23K05881). The authors have no other relevant affiliations or financial involvement with any organization or entity with a financial interest in or financial conflict with the subject matter or materials discussed in the manuscript apart from those disclosed.
No writing assistance was utilized in the production of this manuscript.
Ethical conduct of research
Animal sampling and experimentation were conducted according to institutional and national guidelines for animal ethics.
Open access
This work is licensed under the Attribution-NonCommercial-NoDerivatives 4.0 Unported License. To view a copy of this license, visit http://creativecommons.org/licenses/by-nc-nd/4.0/
Papers of special note have been highlighted as: • of interest; •• of considerable interest
References
- 1. . Cyclostomes (lamprey and hagfish). Handbook of Marine Model Organisms in Experimental Biology. CRC Press, FL, USA, 403–417 (2021). •• Describes the evolutionary and phylogenetically importance of lampreys.
- 2. . Gene expression analysis of lamprey embryos. In: In Situ Hybridization Methods. Giselbert H (Ed.). Humana Press, NY, USA, 263–278 (2015). •• Not only describes the protocol for in situ hybridization on lamprey embryos, but also summarize the methods for adult lamprey maintenance, embryo collection and cultivation.
- 3. . Normal stages of development in the lamprey, Lampetra-Reissneri (Dybowski). Zoolog. Sci. 5(1), 109–118 (1988).
- 4. . The sea lamprey Petromyzon marinus. Cold Spring Harb. Protoc. 2009(1), 405–429 (2009).
- 5. . Structure of the lamprey yolk lipid-protein complex lipovitellin-phosvitin at 2.8 A resolution. J. Mol. Biol. 200, 553–569 (1988).
- 6. . Single-step method of RNA isolation by acid guanidinium thiocyanate-phenol-chloroform extraction. Anal. Biochem. 162, 156–159 (1987).
- 7. The RIN: an RNA integrity number for assigning integrity values to RNA measurements. BMC Mol. Biol. 7, 3 (2006). • Established the RNA integrity number value as a quality assessment metric for RNA.
- 8. . Characterization of somatically-eliminated genes during development of the sea lamprey (Petromyzon marinus). Mol. Biol. Evol. 33, 2337–2344 (2016). • Represents one of the earliest transcriptome analyses of lamprey early-stage embryos.
- 9. Reconstructing the ancestral vertebrate brain using a lamprey neural cell type atlas. bioRxiv
2022.2002.2028.482278 (2022). •• Performed a single-cell transcriptome analysis of the brain of lamprey larvae. - 10. . Transcriptomes of injured lamprey axon tips: single-cell RNA-seq suggests differential involvement of MAPK signaling pathways in axon retraction and regeneration after Spinal Cord Injury. Cells 11, 2320 (2022).
- 11. . Modification of the TRI reagent procedure for isolation of RNA from polysaccharide- and proteoglycan-rich sources. BioTechniques 19, 942–945 (1995).